Dual targeting of FAK and JAK/STAT3 signalling induces persistent inhibition of pancreatic ductal adenocarcinoma progression
Pancreatic ductal adenocarcinoma (PDAC) is an aggressive malignancy with rising incidences and poor prognosis. High susceptibility to metastasis, end stage diagnosis and low response to present therapeutic procedures make it a devastating disease with a 5-year survival below 9% and projected to be the second leading cause of cancer death by 2030 (1). Gemcitabine, alone or in combination, has been used as a first-line treatment for over a decade. Unfortunately, despite several clinical trials focusing on distinct molecular mechanisms, there has been only a marginal improvement in clinical settings (2). Owing to a unique and complex tumour microenvironment (TME), PDAC develops drug resistance via numerous pathways. The substitution of the normal pancreatic parenchyma with desmoplastic TME is an important pathophysiological characteristic of pancreatic cancer. The highly dense TME is composed of immune cells, extracellular matrix (ECM), cancer associated fibroblasts (CAFs), several signalling molecules and tumour cells, and plays a pivotal role in PDAC progression (3). Indeed, stromal cells in the TME have been recognised as an important target to counteract PDAC progression. More specifically, in the past few years, the idea of tumour stroma ablation has been replaced by the concept of tumour stroma remodelling or reprogramming. In other words, treatment approaches should aim at modulating TME in order to increase the sensitivity to therapy (4). To be able to remodel or reprogram TME, however, it is crucial to understand fully the roles played by different TME components in PDAC pathogenesis.
A key role in this process is played by chronic inflammation due to cross talk between the tumour and its microenvironment resulting in alterations of ECM, immune cell recruitment, fibroblast activation and in aberrant signalling, including aberrant cytokine secretion. These, along with other molecular and cellular mechanisms, interdependently affect PDAC aggressivity. The chronic inflammation and cytokine signalling is mediated by Janus Kinases (JAK) which further activate signal transducer and activator of transcription 3 (STAT3) (5,6). Aberrant STAT3 expression has been associated with over 70% of cancers (7). High-STAT3 expression inversely correlates with the overall survival in both human and mouse tumours. It has also been linked to the promotion of cancer cell-mediated CAFs.
In particular, studies have demonstrated that STAT3 activation is imperative for initiation and development of PDAC (8-10) and that STAT3 plays a critical role in the development of PDAC precursors (11) as well as in the establishment of CAFs in a PDAC TME (12). Furthermore, overexpression of STAT3 in tumours plays an important role in acquiring resistance to treatment (13). Taken together, these data suggest that JAK/STAT signalling pathway serves as a potential therapeutic target in PDAC (14), in particular aiming at TME remodelling/reprogramming.
This evidence prompted investigations on the effect of different drugs on activation of JAK/STAT pathway (15) or on STAT3 expression levels and how this could affect TME.
For instance, Nagathihalli et al. demonstrated that a combined therapy of a STAT3 inhibitor and Gemcitabine was able to improve tumour micro vessel density as well as drug delivery without stromal depletion (16), supporting the conclusion that targeting JAK/STAT pathway can represent a successful strategy to remodel the TME. Recently, Jiang et al. investigated how a decrease in stromal density modulates tumour susceptibility to focal adhesion kinase (FAK) inhibition via activation of STAT3 (17).
This study builds on a previous report which indicated that VS-4718, a potent and reversible inhibitor of FAK (FAKi), increases tumour sensitivity to targeted therapies. FAK has been reported to be overactive in PDAC and correlates with TME modulated immune suppression (18). They further reported the existence of mechanisms of resistance to FAK inhibition which allowed tumour re-growth.
By using genetically modified PDAC mouse models such as KPC (p48-Cre, LSL-KrasG12D/wt and p53flox/wt), KPPC (p48-Cre, LSL-KrasG12D/wt and p53flox/flox) and syngeneic transplantable mice models in the latter study (17), Jiang et al. established that STAT3 signalling is involved in mechanism of resistance to FAK inhibition. Hyperactivated STAT3 was reported in tumours displaying poor response to FAK inhibition and was inversely correlated with overall survival, indicating that STAT3 signalling acts as a survival pathway during prolonged FAKi treatment. Also, high phosphorylated STAT3 (pSTAT3) level was confirmed in both the tumour and stroma of KPC and transplantable mouse models.
Furthermore, the authors showed that downregulation of STAT3 using RNA interference both in vivo and in vitro, increases susceptibility to VS-4718 treatment. Importantly, when KPC cells were cocultured with either control (siNC) or STAT3-depleted (siSTAT3) fibroblasts in the presence of FAKi, it was found that loss of pSTAT3 in fibroblasts did not affect the inhibitory effects of FAKi on KPC cells. Taken together, these data suggest that STAT3 in tumour cells, but not in fibroblasts, modulates the response strength to FAKi treatment. Interestingly, the authors observed that inhibiting FAK does not regulate STAT3 signalling directly.
Data revealed that treatment with VS-4718 downregulated both transforming growth factor beta (TGF-β1) and phosphorylated SMAD3 expression in the tumour microenvironment. This was further associated with a decrease in collagen density as well as α-SMA+ CAFs.
In addition, the authors observed that treatment of KPPC mouse models with anti TGF-β antibody resulted in an increase of pSTAT3 levels in PDAC tissues.
Using RNA in situ hybridisation, it was found that α-SMA+ CAFs have an increased expression of TGF-β1 which was consistent with the in vitro mRNA evaluation.
Furthermore, the authors reported that pSMAD3 was inversely correlated with pSTAT3 and positively correlated with FAK, further suggesting that the TGF-β1/SMAD3 signalling pathway might be involved in STAT3 activation in PDAC (Figure 1).
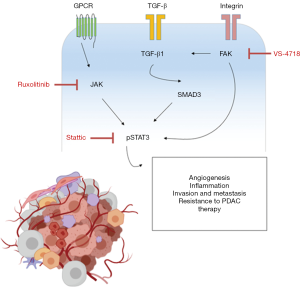
However, FAKi resistance mechanism does not involve non-canonical aspects of the TGF-β1 pathway. Furthermore, SMAD3-dependent JAK1 downregulation post TGF-β1 exposure clearly implies signalling inhibition.
Jiang and colleagues further assessed whether resistance to FAKi could be prevented by simultaneous suppression of both STAT3 signalling and FAK.
Intriguingly, the combination of STAT3 inhibitor Stattic or JAK1 inhibitor Ruxolitinib with VS-4718 in vitro synergistically decreased cancer cell growth specifically in both KPPC and syngeneic mouse models. Also, the efficacy of this combination was unaffected by T cell depletion (CD4+/CD8+).
This clearly validates the conclusion that blocking JAK/STAT3 pathway reduces tumour growth which renders PDAC sensitive to FAKi. Consistently, another study reported STAT3 knockdown resulted in improved sensitivity to Gemcitabine and significant decrease in tumour burden in vivo (19).
Taken together, these findings provide a novel therapeutic approach to target TME in PDAC. Pharmacological agents targeting FAK have already been tested in clinical trials. For instance, VS-4718 was previously under phase 1 trial and administered in combination with both Gemcitabine and Nab-Paclitaxel (NCT02651727) in pancreatic cancer. Currently, another FAKi, Defactinib, is being trialled in PDAC patients (VS-6063, Verastem) in combination with Gemcitabine and Pembrolizumab (NCT02546531) or in combination with Pembrolizumab following chemotherapy as a neoadjuvant and adjuvant treatment for resectable PDAC (NCT03727880).
Overall, Jiang et al. have provided the rationale that can lead to the potentiation of the effect of FAKi involving the modulation of TME, ultimately leading to the development of novel treatment strategies in PDAC.
It would be interesting to test this strategy by combining novel compounds targeting both FAK and STAT3. For instance, we have recently reported that the ABC transporter ABCC3 influences PDAC cell growth by modulating STAT3 activity and regulating apoptosis. Furthermore, pharmacological inhibition with MCI-175, a small compound able to inhibit ABCC3, reduced phosphorylated STAT3 levels in KPC primary cells (20). Interestingly, recent studies show that combined treatment of STAT3 and EGFR might overcome drug resistance in pancreatic cancer cells (21,22).
However, potential complications should be taken into account when designing these strategies. A recent study found that loss of FAK in a subpopulation of CAFs is sufficient to induce increased tumour growth and enhance malignant cell glycolysis (23). These observations have been validated in both human pancreatic and breast cancers with low stromal FAK expression. In the latter study (23), the authors found that loss of FAK in a subpopulation of CAFs causes the enrichment of cytokine signalling pathways that induces an increase of glycolysis in malignant cells. Therefore, the authors unveil a mechanism of tumour cell metabolism that requires the expression of FAK in CAFs (23). It should be noted, however, that FAK has both kinase and scaffold functions and therefore FAK kinase inhibition do not necessarily replicate the phenotype of FAK depletion.
In summary, these findings provide a novel crucial information concerning the tumour-TME interplay and insight in the mechanisms of therapy resistance that will be pivotal in the design of new therapeutic strategies aiming at modulating the TME in order to increase PDAC treatment.
Acknowledgments
The authors acknowledge the infrastructure and staff support provided by Curtin Health Innovation Research Institute and the School of Pharmacy and Biomedical Sciences, Curtin University. PM is supported by the Curtin University Health Sciences Faculty International Research Scholarships.
Funding: This work was supported by Avner Pancreatic Cancer Research and Keith & Ann Vaughan Pancreatic Cancer Fund.
Footnote
Provenance and Peer Review: This article was commissioned by the editorial office, Digestive Medicine Research. The article did not undergo external peer review.
Conflicts of Interest: Both authors have completed the ICMJE uniform disclosure form (available at http://dx.doi.org/10.21037/dmr-20-44). The authors have no conflicts of interest to declare.
Ethical Statement: The authors are accountable for all aspects of the work in ensuring that questions related to the accuracy or integrity of any part of the work are appropriately investigated and resolved.
Open Access Statement: This is an Open Access article distributed in accordance with the Creative Commons Attribution-NonCommercial-NoDerivs 4.0 International License (CC BY-NC-ND 4.0), which permits the non-commercial replication and distribution of the article with the strict proviso that no changes or edits are made and the original work is properly cited (including links to both the formal publication through the relevant DOI and the license). See: https://creativecommons.org/licenses/by-nc-nd/4.0/.
References
- Paternoster S, Falasca M. The intricate relationship between diabetes, obesity and pancreatic cancer. Biochim Biophys Acta Rev Cancer 2020;1873:188326. [Crossref] [PubMed]
- Adamska A, Elaskalani O, Emmanouilidi A, et al. Molecular and cellular mechanisms of chemoresistance in pancreatic cancer. Adv Biol Regul 2018;68:77-87. [PubMed]
- Pothula SP, Pirola RC, Wilson JS, et al. Pancreatic stellate cells: Aiding and abetting pancreatic cancer progression. Pancreatology 2020;20:409-18. [Crossref] [PubMed]
- Neesse A, Bauer CA, Ohlund D, et al. Stromal biology and therapy in pancreatic cancer: ready for clinical translation? Gut 2019;68:159-71. [Crossref] [PubMed]
- Ferrone C, Dranoff G. Dual roles for immunity in gastrointestinal cancers. J Clin Oncol 2010;28:4045-51. [Crossref] [PubMed]
- Jarnicki A, Putoczki T, Ernst M. Stat3: linking inflammation to epithelial cancer - more than a "gut" feeling? Cell Division 2010;5:14. [Crossref] [PubMed]
- Johnson DE, O'Keefe RA, Grandis JR. Targeting the IL-6/JAK/STAT3 signalling axis in cancer. Nat Rev Clin Oncol 2018;15:234-48. [Crossref] [PubMed]
- Scholz A, Heinze S, Detjen KM, et al. Activated signal transducer and activator of transcription 3 (STAT3) supports the malignant phenotype of human pancreatic cancer. Gastroenterology 2003;125:891-905. [Crossref] [PubMed]
- Miyatsuka T, Kaneto H, Shiraiwa T, et al. Persistent expression of PDX-1 in the pancreas causes acinar-to-ductal metaplasia through Stat3 activation. Genes Dev 2006;20:1435-40. [Crossref] [PubMed]
- D'Amico S, Shi J, Martin BL, Crawford HC, et al. STAT3 is a master regulator of epithelial identity and KRAS-driven tumorigenesis. Genes Dev 2018;32:1175-87. [Crossref] [PubMed]
- Fukuda A, Wang SC, Morris JP 4th, et al. Stat3 and MMP7 contribute to pancreatic ductal adenocarcinoma initiation and progression. Cancer Cell 2011;19:441-55. [Crossref] [PubMed]
- Fizazi K, De Bono JS, Flechon A, et al. Randomised phase II study of siltuximab (CNTO 328), an anti-IL-6 monoclonal antibody, in combination with mitoxantrone/prednisone versus mitoxantrone/prednisone alone in metastatic castration-resistant prostate cancer. Eur J Cancer 2012;48:85-93. [Crossref] [PubMed]
- Lee HJ, Zhuang G, Cao Y, et al. Drug resistance via feedback activation of Stat3 in oncogene-addicted cancer cells. Cancer Cell 2014;26:207-21. [Crossref] [PubMed]
- Lin L, Hutzen B, Zuo M, et al. Novel STAT3 phosphorylation inhibitors exhibit potent growth-suppressive activity in pancreatic and breast cancer cells. Cancer Res 2010;70:2445-54. [Crossref] [PubMed]
- Quintás-Cardama A, Verstovsek S. Molecular Pathways: JAK/STAT Pathway: Mutations, Inhibitors, and Resistance. Clin Cancer Res 2013;19:1933-40. [Crossref] [PubMed]
- Nagathihalli NS, Castellanos JA, Shi C, et al. Signal Transducer and Activator of Transcription 3, Mediated Remodeling of the Tumor Microenvironment Results in Enhanced Tumor Drug Delivery in a Mouse Model of Pancreatic Cancer. Gastroenterology 2015;149:1932-1943.e9. [Crossref] [PubMed]
- Jiang H, Liu X, Knolhoff BL, et al. Development of resistance to FAK inhibition in pancreatic cancer is linked to stromal depletion. Gut 2020;69:122-32. [Crossref] [PubMed]
- Jiang H, Hegde S, Knolhoff BL, et al. Targeting focal adhesion kinase renders pancreatic cancers responsive to checkpoint immunotherapy. Nat Med 2016;22:851-60. [PubMed]
- Venkatasubbarao K, Peterson L, Zhao S, et al. Inhibiting signal transducer and activator of transcription-3 increases response to gemcitabine and delays progression of pancreatic cancer. Mol Cancer 2013;12:104. [Crossref] [PubMed]
- Adamska A, Domenichini A, Capone E, et al. Pharmacological inhibition of ABCC3 slows tumour progression in animal models of pancreatic cancer. J Exp Clin Cancer Res 2019;38:312. [Crossref] [PubMed]
- Zhao C, Yang L, Zhou F, et al. Feedback activation of EGFR is the main cause for STAT3 inhibition-irresponsiveness in pancreatic cancer cells. Oncogene 2020;39:3997-4013. [Crossref] [PubMed]
- Dosch AR, Dai X, Reyzer ML, et al. Combined Src/EGFR inhibition targets STAT3 signaling and induces stromal remodeling to improve survival in pancreatic cancer. Mol Cancer Res 2020;18:623-31. [Crossref] [PubMed]
- Demircioglu F, Wang J, Candido J, et al. Cancer associated fibroblast FAK regulates malignant cell metabolism. Nat Commun 2020;11:1290. [Crossref] [PubMed]
Cite this article as: Malhotra P, Falasca M. Dual targeting of FAK and JAK/STAT3 signalling induces persistent inhibition of pancreatic ductal adenocarcinoma progression. Dig Med Res 2020;3:89.